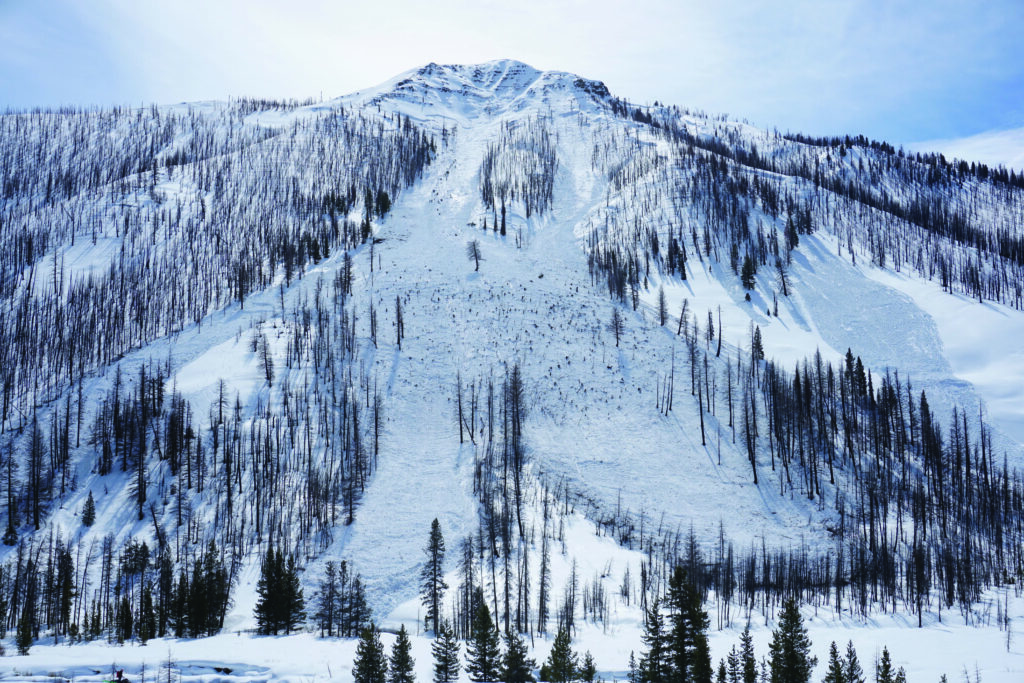
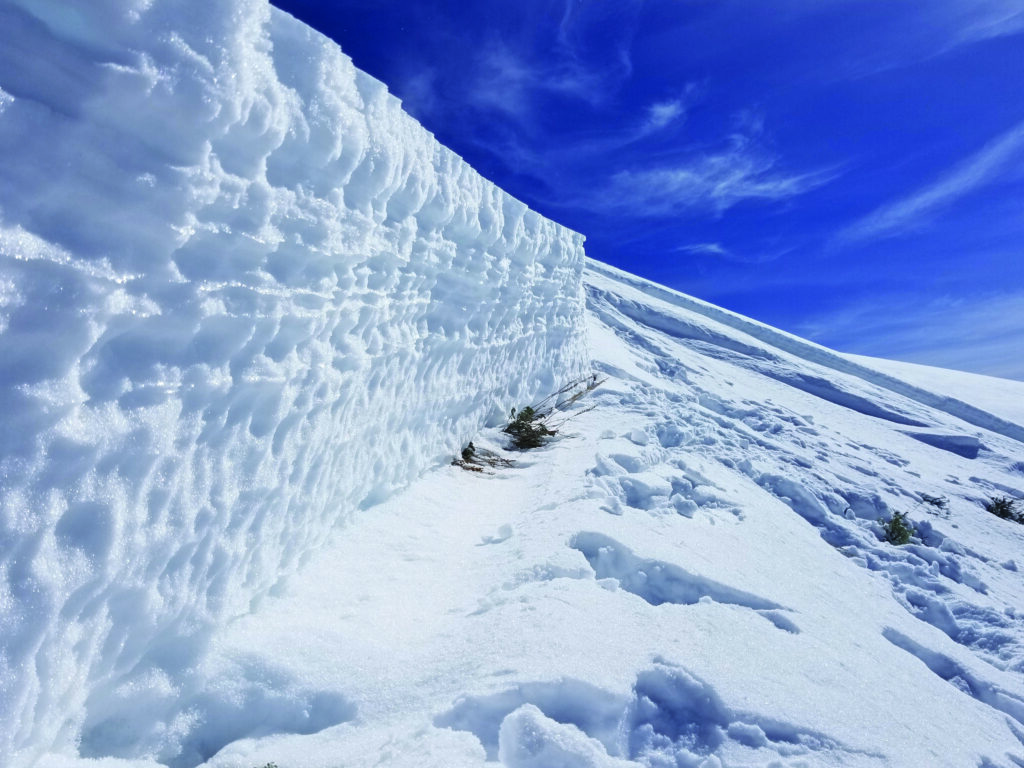
Forecasting for dry and wet avalanches during mixed rain and snow storm events
By Scott Savage, Erich Peitzsch, Simon Trautman, and Ben Vendenbos
Professionals in coastal and some inland mountain ranges regularly face mixed rain-snow events. Professionals in inland ranges frequently deal with persistent slab avalanches failing on old faceted layers buried deep within the snowpack. What happens when you combine these snowpack and weather events? Widespread avalanching involving faceted layers during mixed rain-snow events is rarely observed and is not as well-understood. Last March, USFS Sawtooth Avalanche Center (SAC) staff observed this scenario.
Snowpack History
The SAC’s Wood River Valley (WRV) zone harbored a shallow, weak, snowpack with faceted snow crystals from December (December weak layer) through early January 2018. Below 7500 ft., many slopes were devoid of snow. Upper elevation and alpine starting zones contained snow, but several strong wind events scoured many exposed slopes. From early January through mid-February, light to moderate snowfall interspersed with dry, cool, sunny periods formed a layer of near-surface faceted crystals associated with crusts on some aspects (Figure 1). This layer was subsequently buried on 14 February. A storm on 1-2 March deposited approximately 50 mm (2 in.) of snow water equivalent (SWE), producing an extensive natural avalanche cycle on the December and 14 February weak layers. Additional snowfall from 14-17 March caused another round of natural avalanche activity on the 14 February layer.
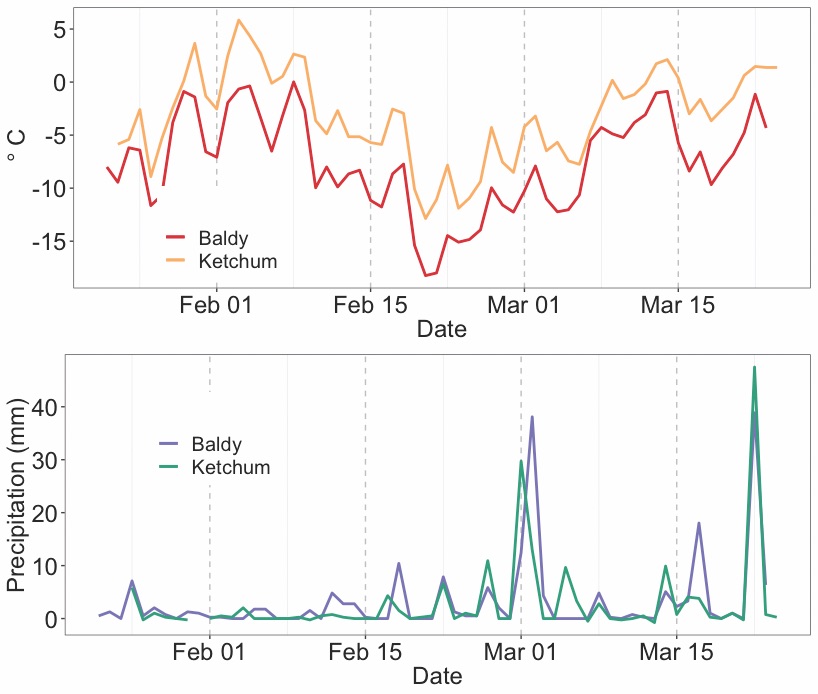
Storm Event
A southwest flow delivered a warm, wet storm system beginning early on 22 March. In the 24 hours following the onset of precipitation, remote weather stations near Ketchum-Sun Valley recorded 45-65 mm (1.75-2.5 in.) of precipitation. Direct observations of surface crusts in the following days indicated rain-snow levels ranged from 7700-8700 ft. during the storm accompanied by moderate to strong south/southeast winds.
Avalanche Activity
Avalanche mitigation at a nearby ski area resulted in multiple intentionally-triggered, D1 loose wet avalanches failing on near-surface crusts on steep slopes between 7550-9000 ft. However, no substantial debris was observed or reported in any large runout zones near the valley floors by sunset on 22 March. Good visibility in the following days allowed for better observations. As expected, widespread D2-D4 natural dry slab avalanches occurred throughout the advisory area at upper elevations above 9000 ft. Crown depths ranged from one to over three meters. The upper elevation events were clustered on west, north, and east aspects. This natural activity was present in much of the advisory area and was most pronounced in the Pioneer Mountains of the WRV zone. Here, widespread 60-105 cm deep, D2-D3 natural slab avalanches also released in middle elevation starting zones between 7500-8900 ft. (Figures 2,3). This middle elevation activity occurred on west, north, and east aspects but was more narrowly focused on northwest aspects. Many slides occurred in paths where activity has not been observed in the previous 30 years (personal communication: Abromeit, Bachman, Bingham, Gardiner, Kellam). The widespread, natural middle elevation activity was confined to a relatively small area—a few drainages—in the WRV zone. The observed avalanches at middle elevations failed on large (2-3 mm) faceted crystals above an ice crust about 20-40 cm above the ground (14 February layer, Figure 4). The uppermost regions of the starting zones held dry surface snow and did not appear to receive any rain. However, based on field observations, rain fell in substantial portions of many of the starting zones for at least part of the precipitation event. In the lower parts of the starting zones and in the avalanche tracks, the snowpack above the failure layer was much thinner; free water from rainfall had percolated through the slab to the failure layer and bed surface. At one observed avalanche site, the slab changed from a completely dry, 80 cm thick snow mass at the top of the crown to a thoroughly wet, 20 cm thick slab 100 vertical meters downslope.
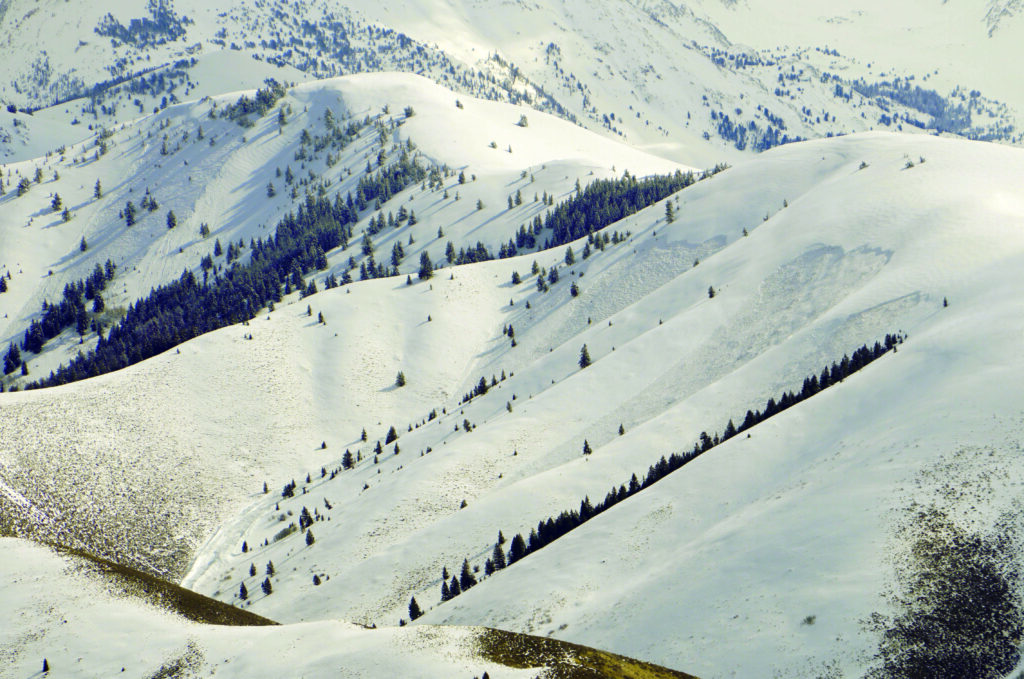
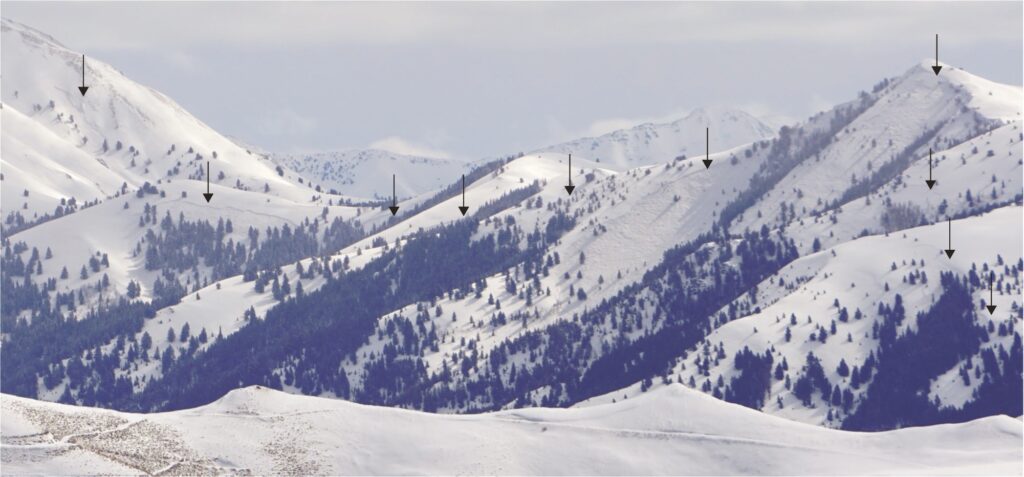
Cause of Elevation Dependency within the WRV Zone
In exposed upper elevation terrain, the combination of existing snowpack structure, heavy snowfall, and extensive wind-transported snow caused the observed dry slab avalanche activity. At elevations that received only rain during the precipitation event, isolated wet loose avalanches released. The snowpack at these lower elevations was quite thin, and water from previous warm weather and rain events had eroded the snowpack or previously wet all layers. Given the temperature and precipitation conditions, we present three possible mechanisms that may explain the widespread middle elevation avalanche activity (Figure 5):
- Loading the snowpack: Snowfall and wind-transported snow overloaded the upper reaches of the starting zone and caused fracture, independent of the water percolating down to the weak layer lower in the starting zones.
- Free water weakening: Rainfall percolating through the snowpack in lower portions of the starting zones stressed or weakened the slopes, promoting fracture and subsequent slope failure.
- Changing slab properties: Snowfall accumulated in portions of the starting zones before rain fell on top of and wet the dry snow, changing the slab properties enough to induce fracture.
Given the uncertainty associated with rain-on-snow induced slab avalanches failing on persistent weak layers, we see a need for synthesizing observations and insight from this case study into a hypothesis. We suggest that a combination of the three stated mechanisms—and possibly other mechanisms not yet observed or characterized—caused the fractures. This hypothesis is consistent with the observed elevation dependency of the low frequency, middle elevation activity during this storm (see sidebar for additional discussion).
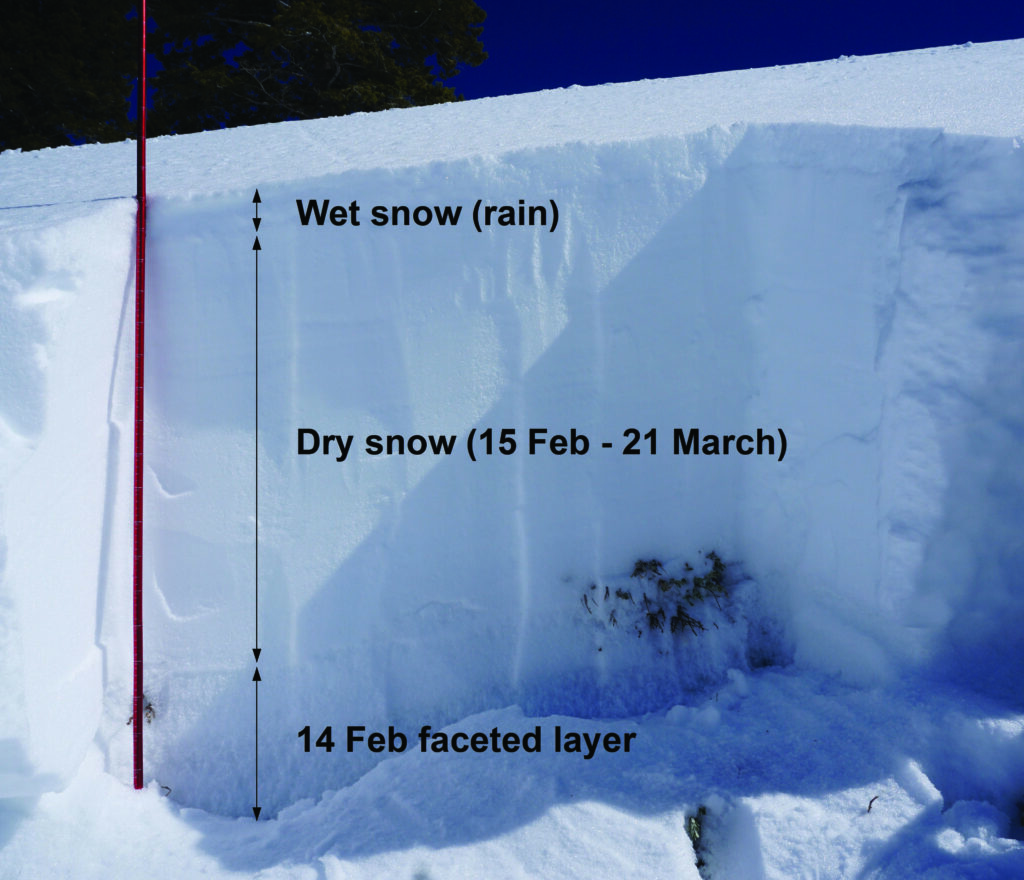
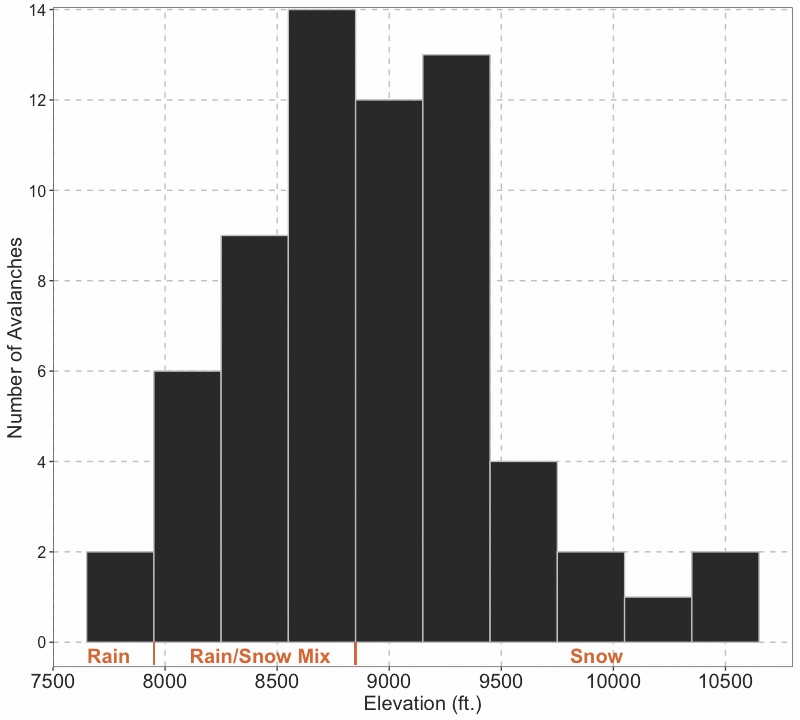
Aspect, Spatial, and Elevation Patterns
The frequency of middle elevation slab releases on northwest aspects in a relatively small area in the Pioneer Mountains was notably different than crowns observed above the rain line. Several factors may help explain the observed patterns:
- Differences in snowpack depth and structure dependent on aspect and elevation: Strong northwest wind events in December and January scoured snow from many exposed northwest-facing slopes, resulting in a thinner overall snowpack and enhanced faceting in early February on those aspects.
- Drainage orientation: The affected drainages are oriented generally southwest to northeast, resulting in a higher proportion of northwest and southeast-facing slopes in the impacted area.
- Solar effects: The snowpack had dramatically thinned or melted on aspects more directly-oriented to the sun.
- Wind transported snow: South to southeast winds blew during much of the storm event, focusing wind-loading on west, northwest, north, and northeast aspects
- Previous avalanche activity: The 2 March storm event produced widespread natural activity, eliminating the 14 February weak layer on some slopes.
Substantial uncertainty exists as to why more middle elevation, north and northeast-facing slopes in the WRV zone did not release.
Forecasting Implications
Extensive slab avalanching occurred in a single mountain range near the rain-snow line in a narrow elevation band, on a narrow range of aspects, over a relatively short time period. Precipitation type, amounts, and pre-existing snowpack structure produced the rarely-observed avalanche activity in this area. Several factors contributed to the difficulty of forecasting this avalanche cycle:
- Precipitation models under-predicted precipitation by a factor of 2.
- Detailed snowpack structure observations were limited by the regional operational scale.
- A precipitation threshold appeared to be exceeded in a limited geographic area; widespread natural activity was confined to the end of the precipitation event and only where over ~2 in. of precipitation fell.
- Staff had little to no experience observing or forecasting previous, similar events.
Communication Implications
In North America, many operations use a conceptual model of avalanche hazard (CMAH) (Statham et al., 2017) as a general framework for assessing and forecasting avalanche hazard. The regional forecasting community and individual operations develop decision trees and communication platforms based on the CMAH. These tools work well in the vast majority of scenarios when transitions between avalanche problems are clear; graphics and text describing avalanche location, likelihood, and size sequentially follow the avalanche problem type. However, wet and dry snow and avalanches occur as a continuum, and scenarios not easily sorted into discrete categories (e.g. problems) occur (Figure 6). The effects of dramatic spatial and temporal changes associated with events like the one presented in this article will stress any discrete sorting platform, despite the number of avalanche problem types employed.
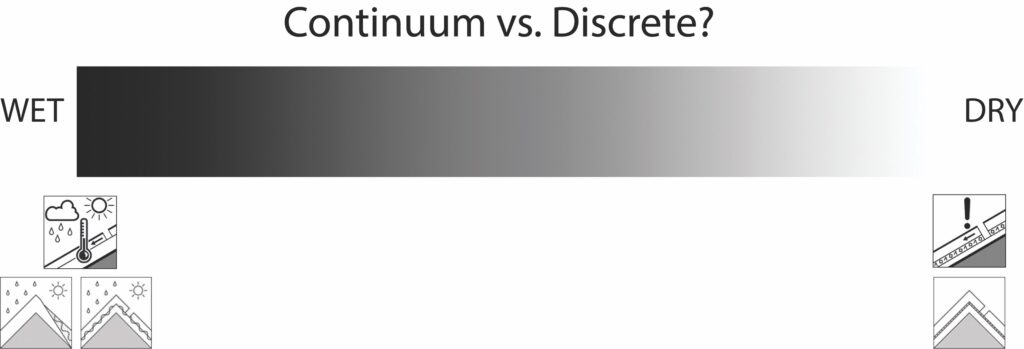
The discrete boxes (avalanche problems) are adequate for sorting or categorizing snow and avalanches the vast majority of the time, but how should we communicate when a discrete sorting approach doesn’t work perfectly? One option is to address the uncertainty. Although considering and addressing uncertainty is paramount in avalanche hazard forecasting and public communication (LaChapelle, 1980; Jamieson et al. 2015), public avalanche forecasts do not graphically display it. One way to mitigate this issue is to describe the uncertainty associated with the avalanche problem illustration in the product text. The caveat is that some consumers may view textual admissions of uncertainty as “indicators of ignorance” instead of actionable information (Lewandowski et al. 2015).
We suggest developing communication tools, techniques, and procedures that better handle a continuum of avalanche problems—especially wet-dry—and can facilitate communicating uncertainty in selecting the primary avalanche problem type. While current communication products work well for “typical” avalanche conditions, improvements may help to communicate dangerous, infrequent events in a consistent manner.
Future Work
Professionals in coastal and some inland mountain ranges regularly face mixed rain-snow events. Southern and central Idaho have not experienced these events frequently, but climate models suggest a higher frequency in the future due to a changing climate (Lazar and Williams, 2008; McCabe et al., 2007; Musselman et al., 2018). We encourage future work investigating wet snow avalanches and developing professional and public communication tools and platforms to handle scenarios as we have described in this article.
References
Abromeit D., Bachman K., Bingham R., Gardiner S., Kellam J. Personal Communication. 2012-2018.
Jamieson B, Haegeli P, Statham G (2015) Uncertainty in snow avalanche risk assessments. In: Proceedings of GEO Que´bec 2015, Que´bec City. 20–23 September 2015.
LaChapelle E (1980) The fundamental processes in conventional avalanche forecasting. Journal of Glaciology. 26(94):75–84.
Lazar, B. and Williams, M., 2008. Climate change in western ski areas: Potential changes in the timing of wet avalanches and snow quality for the Aspen ski area in the years 2030 and 2100. Cold Regions Science and Technology, 51(2-3): 219-228.
Lewandowsky, S., Ballard, T., & Pancost, R. D. (2015). Uncertainty as knowledge. Philosophical Transactions of the Royal Society A: Mathematical, Physical and Engineering Sciences, 373(2055).
McCabe, G.J., Hay, L.E. and Clark, M.P., 2007. Rain-on-Snow Events in the Western United States. Bulletin of the American Meteorological Society, 88(3): 319-328.
Musselman, K.N., Lehner, F., Ikeda, K., Clark, M.P., Prein, A.F., Liu, C., Barlage, M. and Rasmussen, R., 2018. Projected increases and shifts in rain-on-snow flood risk over western North America. Nature Climate Change, 8, 808-812.
Statham, G., Haegeli, P., Greene, E., Birkeland, K., Israelson, C., Tremper, B., Stethem, C., McMahon, B., White, B. and Kelly, J., 2017. A conceptual model of avalanche hazard. Natural Hazards, 90(2): 663-691.
Savage, S., Peitzsch, E., Trautman, S., VandenBos, B. 2018. Forecasting for dry and wet snow avalanches during mixed rain and snow storm events. In: Proceedings of the 2018 International Snow Science Workshop, Innsbruck, Austria, 8-12 October 2018. pp. 1040-1044.
About the Authors
Scott Savage is the Director of the USFS Sawtooth Avalanche Center in Ketchum, Idaho. He considers each day that he learns more than he forgets to be a success.
Erich Pietzsch currently studies avalanches with chainsaws and drones.
Simon Trautman works for the USFS National Avalanche Center and is the acting Director of NWAC for the 2018–2019 season.
Ben VandenBos is a Forecaster at the USFS Sawtooth Avalanche Center. He has come to realize that his skiing compulsion is a feature, not a bug.